THE LATEST
The effectiveness of stimulating nanomagnets via infrared lasers: A quantum leap or a bridge too far?
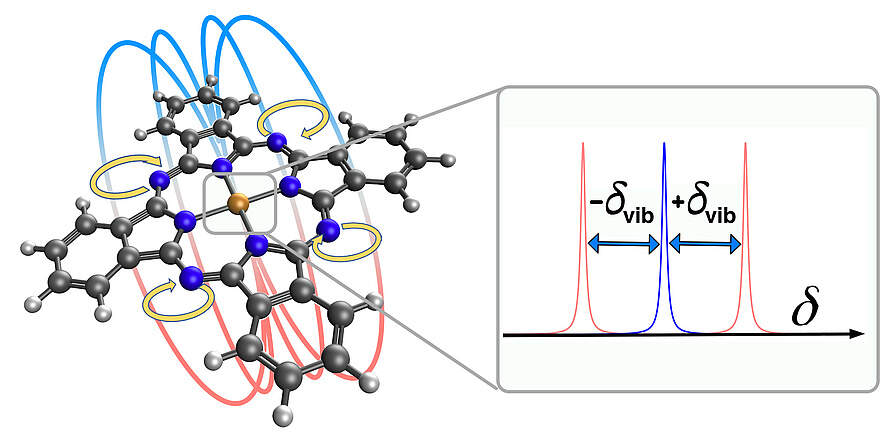
The recent findings from Graz University of Technology (TU Graz) have sparked a lively debate within scientific circles about a pioneering methodology developed by a team of physicists at the institute. Their research focuses on using infrared light pulses to stimulate nanomagnets, offering a promising potential application in quantum computer circuits. However, skeptics have started to question the practicality and feasibility of this innovative concept amidst the excitement of scientific progress.
At the heart of this paradigm-shifting proposal lies the core hypothesis that certain molecules, when stimulated by infrared radiation, exhibit intricate vibrational responses that give rise to minute magnetic fields, operating on the nanometer scale. The leading figure behind this innovative inquiry, Andreas Hauser from the Institute of Experimental Physics at TU Graz, draws on the established understanding that molecular oscillations induced by infrared light can engender magnetic fields, underpinned by the charged nature of atomic nuclei in response to particle movement.
However, the proposed application of this principle in quantum computer circuits invites a level of skepticism that underscores the complexity and uncertainty underpinning this audacious venture. The theoretical framework posits that the manipulation of infrared light could potentially facilitate precise control over the strength and direction of these magnetic fields, effectively transforming the molecules into ultra-precise optical switches for potential integration into quantum computer circuits.
The methodology employed in this groundbreaking research, as derived from calculations performed on supercomputers, harnesses modern electron structure theory to meticulously chart the behavioral patterns of phthalocyanine molecules under the influence of circularly polarized infrared light. The results purport to showcase the rapid generation of localized magnetic fields when these molecules are subjected to helically twisted light waves.
The concept of leveraging these molecular magnetic fields for potential use in quantum computer circuits beckons a pivotal question—can the intricacies of molecular responses to infrared stimuli truly be harnessed as a reliable and scalable foundation for advanced quantum computing systems? A healthy dose of skepticism arises from the complexity inherent in translating theoretical simulations into practical, real-world applications within quantum computing environments, particularly about the consistency and reproducibility of the proposed molecular magnetic field manipulation.
The upcoming experimental phase is seen as a crucial test to validate the creation of molecular magnetic fields through controlled methods. This stage promises to provide further insight into the feasibility and truth of these groundbreaking claims. The effort to computationally model the interaction between deposited phthalocyanines, support materials, and infrared light is a pivotal turning point, where the theoretical framework's success or failure will be determined.
The research is very important, but we need to be careful and critical in our approach. The scientific community is waiting for the results of the upcoming experiments, and there is a big debate about whether using molecular magnetic fields in quantum computer circuits is practical and reliable.