THE LATEST
Flatiron Institute announces new detectable gravitational wave source from collapsing stars, as predicted by simulations
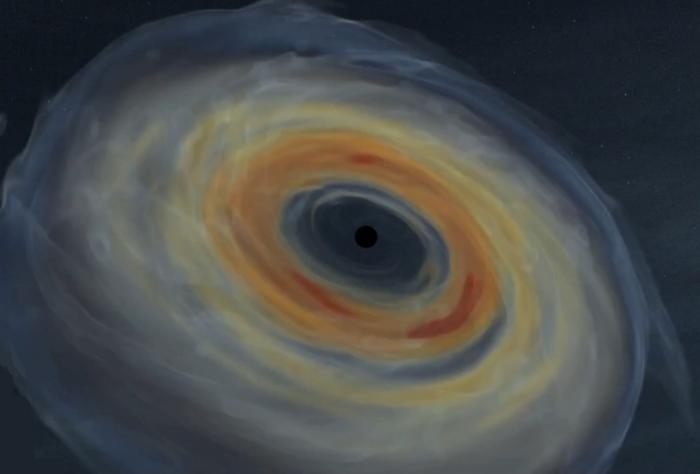
In a recent study, researchers from the Flatiron Institute present simulations indicating that detectable gravitational waves could originate from the cataclysmic collapse of massive spinning stars. If proven true, this revelation could potentially revolutionize our understanding of the cosmos and the nature of black holes. However, these unprecedented claims have left many in the scientific community skeptical and cautious about embracing such paradigm-shifting assertions.
The study's bold assertions are based on the utilization of cutting-edge computational simulations. The simulations purportedly demonstrate the emergence of detectable gravitational waves following the dramatic deaths of rapidly rotating stars, offering a tantalizing prospect of expanding the horizons of gravitational wave astronomy.
While the potential implications of these findings are undeniably profound, the underlying fragility and speculative nature of simulations render the research subject to intense scrutiny. It is imperative to acknowledge that simulations, no matter how sophisticated, are inherently simplifications of complex physical phenomena.
Lead researcher Ore Gottlieb, a research fellow at the Flatiron Institute’s Center for Computational Astrophysics, is assertive in his claims that these gravitational waves could be detectable with instruments such as LIGO, the Laser Interferometer Gravitational-Wave Observatory. The predictions even suggest that current datasets might already contain evidence of these elusive signals.
However, the scientific community remains skeptical about the feasibility and robustness of these simulations. The boldness of the claims – presenting the possibility of fundamentally altering our understanding of black holes and the inner workings of collapsing stars – invites cautious contemplation.
The study's acknowledgements of the limitations of simulations reinforce the need for healthy skepticism. Gottlieb himself admits the challenge of capturing the variability and complexity of massive stars' collapse through simulations, illustrating the inherent uncertainties and assumptions in the endeavor.
Moreover, the proposal for detecting these gravitational waves raises pertinent questions about the capability of existing instruments and the potential biases in interpreting observational data. The complexities and subtleties of detecting elusive signals from celestial events demand a rigorous and vigilant approach that is far from guaranteed by relying solely on the outcomes of computational simulations.
While the study’s attempts to shed light on hitherto unexplored aspects of astrophysics are commendable, the scientific community should approach these claims with skepticism and scrutiny. The steadfast reliance on simulations demands a thorough validation process that adheres to the rigors of empirical evidence and observational corroboration.
As we confront these grand claims stemming from meticulously designed simulations, it becomes paramount to exercise caution and temper our enthusiasm with the sobering reality of the complexities inherent in modeling complex astrophysical phenomena. True scientific progress can only be achieved through the meticulous testing and validation of hypotheses, especially when they are based on the speculative outcomes of computational simulations.