INDUSTRY
Lymphotactin shape-changes revealed using Tungsten
A team of researchers from the University of Wisconsin are using NCSA's Tungsten supercomputing system to investigate lymphotactin, a protein manufactured by progenitor T-cells in the human body. Lymphotactin has been linked to autoimmune diseases and to the rejection of transplanted organs. Led by Qiang Cui, an assistant professor in the chemistry department, they are simulating the impact that temperature and salt concentration have on lymphotactin's structure. Protein structure can be influenced by such factors in the surrounding solvent, and it heavily influences protein function. Lymphotactin, for example, is thought to stimulate the immune system by binding to specific protein-coupled receptors on immune-system cells. It can only do that if it has taken on specific shapes.
Contemporary experimental studies of the protein using nuclear magnetic resonance imaging tend to change temperature and salt concentration of lymphotactin's environment at the same time. In a paper published in the Journal of the American Chemical Society in July 2006, the Wisconsin team's molecular dynamics simulations treated these variables separately. They found that chloride from the salt in the solvent is more attracted to most sections of the protein at higher temperatures. In what is known as the "C-terminal helical region," however, both chloride and sodium distributions are higher at lower temperatures. The team also found that the C-terminal helix partially melts at higher temperatures, regardless of the amount of salt, whereas another region begins to form a helical structure at higher salt concentrations. "These explicit solvent simulations on the order of 70 nanoseconds would not be possible without the generous support of computational resources from NCSA. The user-friendly infrastructure of NCSA has not only provided the facility for carrying out the molecular simulations required by our projects but also stimulated us to pursue more challenging problems in the biophysical and biomedical area," says Cui. These simulations only reveal the initial stages of lymphotactin's structural change, though they demonstrate the significant impact that environmental variables can have. The team is now in the process of performing more extensive simulations for lymphotactin's other structural form. "Nearly every single backbone hydrogen bond has to be broken in the structural conversion process," says Cui. "It's truly remarkable that these striking changes -- along with the tendency to dimerize [or bond two identical copies of the protein to one another] -- are facilitated by something as simple as temperature and salt." The team also recently published NCSA-based simulations of the gating pathways of mechanosensitive channels of large conductance in two bacteria using a finite element method, as well as simulations showing the activation mechanism of an allosteric protein.
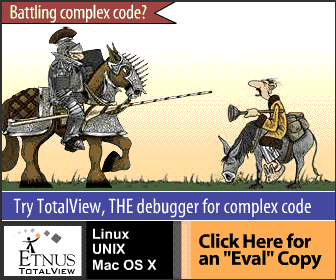