INDUSTRY
Wang identifies new sources of turbulence in spherical tokamaks
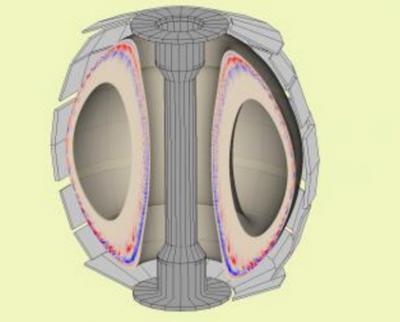
For fusion reactions to take place efficiently, the atomic nuclei that fuse together in plasma must be kept sufficiently hot. But turbulence in the plasma that flows in facilities called tokamaks can cause heat to leak from the core of the plasma to its outer edge, causing reactions to fizzle out.
Researchers at the U.S. Department of Energy's (DOE) Princeton Plasma Physics Laboratory (PPPL) have for the first time modeled previously unsuspected sources of turbulence in spherical tokamaks, an alternative design for producing fusion energy. The findings, published online in October in Physics of Plasmas, could influence the development of future fusion facilities. This work was supported by the DOE Office of Science.
Spherical tokamaks, like the recently completed National Spherical Torus Experiment-Upgrade (NSTX-U) at PPPL, are shaped like cored apples compared with the mushroom-like design of conventional tokamaks that are more widely used. The cored-apple shape provides some distinct characteristics for the behavior of the plasma inside.
The paper, with principal research physicist Weixing Wang as lead author, identifies two important new sources of turbulence based on data from experiments on the National Spherical Torus Experiment prior to its upgrade. The discoveries were made by using state-of-the-art large-scale supercomputer simulations. These sources are:
* Instabilities caused by plasma that flows faster in the center of the fusion facility than toward the edge when rotating strongly in L-mode -- or low confinement -- regimes. These instabilities, called "Kelvin-Helmholtz modes" after physicists Baron Kelvin and Hermann von Helmholtz, act like wind that stirs up waves as it blows over water and are for the first time found to be relevant for realistic fusion experiments.
Such non-uniform plasma flows have been known to play favorable roles in fusion plasmas in conventional and spherical tokamaks. The new results from this study suggest that we may also need to keep these flows within an optimized level in spherical tokamaks.
* Trapped electrons that bounce between two points in a section of the tokamak instead of swirling all the way around the facility. These electrons were shown to cause significant leakage of heat in H-mode -- or high-confinement -- regimes by driving a specific instability when they collide frequently. This type of instability is believed to play little role in conventional tokamaks but can provide a robust source of plasma turbulence in spherical tokamaks.
Most interestingly, the model predicts a range of trapped electron collisions in spherical tokamaks that can be turbulence-free, thus improving the plasma confinement. Such favorable plasmas could possibly be achieved by future advanced spherical tokamaks operating at high temperature.
Findings of the new model can be tested on the NSTX-U and will help guide experiments to identify non-traditional sources of turbulence in the spherical facility. Results of this research can shed light on the physics behind key obstacles to plasma confinement in spherical facilities and on ways to overcome them in future machines.